The simple remedy of glasses paints a rosy picture of myopia, when in actuality there can be serious sequalae of a long eye. The potentially blinding structural complications of myopia can be ascribed to the mechanical strain in the eye’s posterior segment. Specifically, there is thinning of the retina in the equatorial region and the sclera and choroid posteriorly1. Even in the absence of ocular pathology, best corrected visual acuity can be suboptimal in long myopic eyes2. This can be due to higher order optical aberrations or processing dysfunctions downstream from the photoreceptors3. Adding more powerful lenses to diverge light further evidently does not come without consequences. Myopic sequalae accounts for 6% to 33% of cases of low vision in parts of the world4,5. Tideman and colleagues highlighted the gravity of axial length changes in relation to permanent myopia-associated visual impairment6. Their findings parallel the increasing concern of myopia’s public health impact globally.
A Stretched Retina
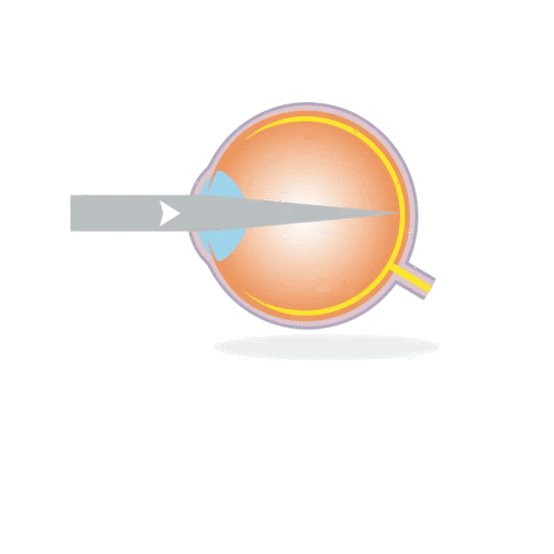
Myopia can begin in early school age and slow its progression in the latter teen years. This general trend has exceptions – refractive error in highly myopic eyes can progress even later into life, but axial growth rates tend to settle around 20 years of age7. That leaves a large latency between the halting of elongation and the presence of posterior segment changes in adulthood. These changes can appear at the levels of the vitreous, peripheral and central retina, and choroid. Some findings that can affect vision, either directly or indirectly, include posterior vitreous detachment, peripheral retinal degeneration, and myopic maculopathy8.
The earlier syneresis of the vitreous humor in myopic eyes can lead to posterior vitreous detachments at younger ages9. Vitreous liquefaction can be a visual nuisance when the clumps cast shadows onto the retina, but the true problem is the vitreoretinal tractional points. Retinal tears and rhegmatogenous retinal detachments are frequently secondary to posterior vitreous detachments and increasingly associated with longer axial lengths10,11.
Lattice degeneration is one of the most common peripheral retinal changes seen in myopic eyes, with higher prevalence with increased axial length12. Its etiology seems to be related to reduced retinal blood flow in the thinned tissues. It is undetermined which is the actual version of the physiology - the reduced circulation leading to thinner retina or the opposite13.
Myopic maculopathy involves the posterior pole and encompasses a group of findings including fundus tessellation, chorioretinal atrophy, lacquer cracks, neovascularization, and posterior staphyloma14. Whereas the other complications of myopia cause acute vision loss, changes at the macula generally occur slowly over a long duration of myopia. Existing myopic maculopathy can continue to worsen with time, especially in the presence of a posterior staphyloma15. Chorioretinal atrophy and fundus tessellation already present in childhood may be an early marker of more advanced myopic maculopathy later in life16,17. The prevalence of myopic maculopathy is well known to be increased with higher axial lengths.
Millimeters Matter
Long axial length is a shared feature in myopic complications, but to understand abnormally long eyes, norms should be explored. Values from emmetropes serve as a reference group well, as myopia should not be considered normal. One-tenth of a millimeter of growth per year in childhood seems to be well-compensated by the eye, as children could remain emmetropic at this rate18. How about axial length norms? There are many epidemiological studies that have gathered data on axial length of children with mixed refractive statuses. However, when considering emmetropes exclusively, the data is limited. Fledelius and colleagues found emmetropic children in Denmark to have an average axial length between 22 mm and 24 mm from 6 to 14 years of age19. Axial length has ethnicity and gender-dependent differences as well, so further studies in a multiracial population can give a fuller picture of axial length norms in school-aged children. Nevertheless, given that high myopia is generally agreed upon as axial lengths greater than 26 mm, the discrepancy of eye length in emmetropia and high myopia is apparent.
Rates of axial length change can be tricky to follow because of the nonlinear growth rates throughout childhood18,20. Centile ranges for axial length values are being developed as a promising reference set. It can be regarded as an “eye growth chart” analogous to a height or weight chart at the pediatrician’s office. Where a child’s axial length is within the representative data can give insight to risk for developing high myopia. These curves have already been modeled in China21 and Europe22, but the their application may be limited to their unique regional context. More databases are needed to prepare a reliable, predictive algorithm for myopia development. An optimal growth curve would include multiple ethnicities and a broad range of ages preceding kindergarten to the end of high school years. Mixed refractive errors are more illustrative of the general population, but axial lengths of emmetropes at different time points can also be valuable benchmarks. Though no centile chart can account for intra-individual changes that occur through childhood, a myopia centile chart will be in advancement for surveillance of natural history and to eventually track the efficacy of myopia control treatments.
Until this ambitious curve is established, the novel idea of a risk score is emerging as an adjunct guide in myopia progression management. Because axial length is undeniably only one piece of a larger puzzle, Tideman and colleagues proposed a risk score including other ocular and environmental considerations. Parental myopia, activities, and ethnicity all had some predictive value on axial elongation23. Elaborating on this concept with multilevel modeling can better estimations of effects 24. Refined receiver operating characteristic curves for predicting myopia may be nigh.
To move out from the backdrop of curves and scores, in short, abnormal axial growth is the chief problem with myopia. It is the biomechanical elongation of the eyes’ tissues that leads to the functional consequences later in life. Tideman clearly stratified the frequency of myopia-related visual impairment as a function of axial length. Eyes longer than 28 mm had an astonishing 11 to 24 times higher risk for visual impairment than eyes shorter than 24 mm18. Consider that in relation to the emmetropic teenagers of Denmark who do not need to face this possibility19. Also in Tideman’s study, spherical equivalent power did not always have a consistent association6. This echoes other work that did not always find a linear relationship between power and axial length across age groups18. The majority of the large myopia trials aptly tag axial elongation as a primary outcome measure to assess efficacy and change. In clinical practice, axial length should be measured as a regular part of myopia management to recognize trajectory and intervene appropriately.

Dr. Tiffany Yanase Park graduated magna cum laude from Southern California College of Optometry and completed a pediatric optometry residency at Children’s Hospital Colorado. Dr. Yanase Park now works as part of a university-based pediatric ophthalmology department in Southern California.
References
-
Jonas JJ et al. Advances in myopia research anatomical findings in highly myopic eyes. Eye Vis (Lond). 2020 Sep2;7:45.
-
Jong M et al. Reduced vision in highly myopic eyes without ocular pathology: the ZOC-BHVI high myopia study. Clin Exp Optom. 2018 Jan;101(1):77-83.
-
Jaworski A et al. Altered visual sensitivity in axial high myopia: a local postreceptoral phenomenon? Invest Ophthalmol Vis Sci. 2006 Aug;47(8):3695-702.
-
Buch H et al. Prevalence and causes of visual impairment according to World Health Organization and United States criteria in an aged, urban Scandinavian population: the Copenhagen City Eye Study. Ophthalmology. 2001 Dec;108(12):2347-57.
-
Xu L et al. Causes of blindness and visual impairment in urban and rural areas in Beijing: The Beijing Eye Study. Ophthalmology. 2006 Jul;113(7):1134.e1-11.
-
Tideman JWL et al. Association of Axial Length With Risk of Uncorrectable Visual Impairment for Europeans With Myopia. JAMA Ophthalmol. 2016 Dec 1;134(12):1355-1363.
-
Lee JTL et al. Progression and Longitudinal Biometric Changes in Highly Myopic Eyes. Invest Ophthalmol Vis Sci. 2020 Apr 9;61(4):34.
-
Jagadeesh D et al. Posterior segment conditions associated with myopia and high myopia. Clin Exp Optom. 2020 Nov;103(6):756-765.
-
Hayashi K et al. Posterior vitreous detachment in highly myopic patients. Invest Ophthalmol Vis Sci. 2020 Apr 9;61(4):33.
-
Mitry D et al. The predisposing pathology and clinical characteristics in the Scottish retinal detachment study. Ophthalmology. 2011 Jul;118(7):1429-34.
-
Crim N et al. Myopia as a risk factor for subsequent retinal tears in the course of a symptomatic posterior vitreous detachment. BMC Ophthalmol. 2017;17:226.
-
Chen DZ et al. Peripheral retinal changes in highly myopic young Asian eyes. Acta Ophthalmol. 2018 Nov;96(7):e846-e851.
-
Grudzińska E, Modrzejewska M. Modern Diagnostic Techniques for the Assessment of Ocular Blood Flow in Myopia: Current State of Knowledge. J Ophthalmol. 2018; 2018: 4694789.
-
Ohno-Matsui K et al. International photographic classification and grading system for myopic maculopathy. Am J Ophthalmol. 2015 May;159(5):877-83.e7.
-
Hayashi K et al. Long-term pattern of progression of myopic maculopathy: a natural history study. Ophthalmology. 2010 Aug;117(8):1595-611, 1611.e1-4.
-
Wong YL et al. Longitudinal Changes in Disc and Retinal Lesions Among Highly Myopic Adolescents in Singapore Over a 10-Year Period. Eye Contact Lens. 2018 Sep;44(5):286-291.
-
Yokoi T et al. Peripapillary diffuse chorioretinal atrophy in children as a sign of eventual pathologic myopia in adults. Ophthalmology. 2016; 123: 1783–1787.
-
Mutti D et al. Refractive error, axial length, and relative peripheral refractive error before and after the onset of myopia. Invest Ophthalmol Vis Sci. 2007 Jun;48(6):2510-9.
-
Fledelius HC et al. Juvenile eye growth, when completed? An evaluation based on IOL-Master axial length data, cross-sectional and longitudinal. Acta Ophthalmol. 2014;92(3):259-64.
-
Rozema J et al. Axial Growth and Lens Power Loss at Myopia Onset in Singaporean Children. Invest Ophthalmol Vis Sci. 2019 Jul;60(8):3091-3099.
-
Sanz Diez P et al. Growth curves of myopia-related parameters to clinically monitor the refractive development in Chinese schoolchildren. Graefes Arch Clin Exp Ophthalmol. 2019 May;257(5):1045-1053.
-
Tideman JWL et al. Axial length growth and the risk of developing myopia in European children. Acta Ophthalmol. 2018 May;96(3):301-309.
-
Tideman JWL et al. Environmental Risk Factors Can Reduce Axial Length Elongation and Myopia Incidence in 6- to 9-Year-Old Children. Ophthalmology. 2019 Jan; 126(1):127-136.
-
Xie Z et al. Prevalence of myopia and associated risk factors among primary students in Chongqing: multilevel modeling. BMC Ophthalmol. 2020 Apr 15;20(1):146.
-
Enthoven CA et al. Interaction between lifestyle and genetic susceptibility in myopia: the Generation R study. Eur J Epidemiol. 2019 Aug;34(8):777-784.